Acousto-Optic Modulator (AOM): Altering Light With Sound
introduction: Acousto-Optic Modulator
Optical setups require extreme precision, and researchers must often implement modulators to adjust the frequency, amplitude, wavelength, and direction of their laser beam. Acousto-Optic Modulator (AOM) uses a sound wave to modulate an incoming beam of light to optimize optical systems and devices. The exiting beam typically diffracts at an angle and carries a different intensity than the input beam. AOM are also able to accommodate a wide variety of bandwidths based on the speed of the acoustic (sound) wave. Researchers are able to control the properties of the exit beam by manipulating the intensity, frequency, phase, polarization, and deflection of the input sound wave. Acousto-optic modulators are used in regenerative amplifiers, Q-switching, mode locking of ultrafast lasers, and more.
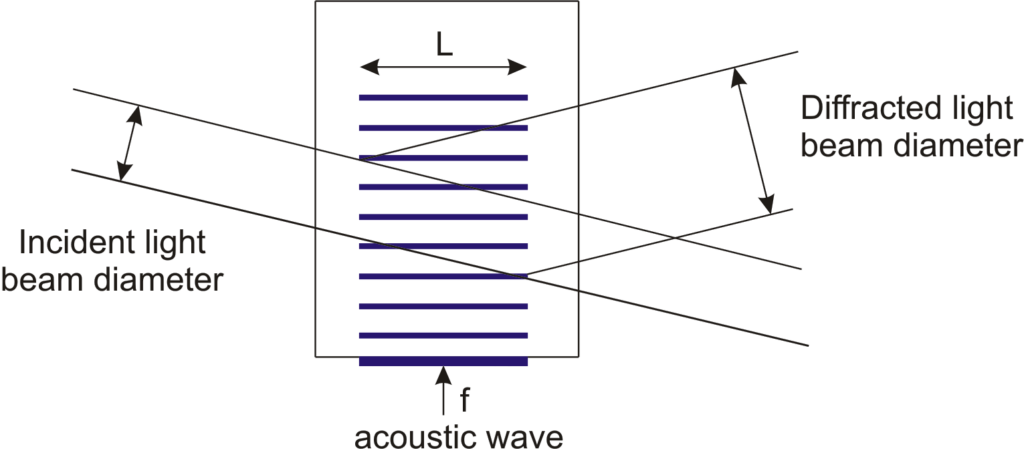
How Does an Acousto-Optic Modulator Work?
Acousto-optic modulator typically uses a transparent crystal or piece of glass as a medium for light propagation. An electric signal then drives an attached transducer to vibrate, therefore generating a sound wave within the cell. This sound wave induces changes in density within the medium, and consequently periodic changes in the refractive index. We can think of these as a type of grating through which the incoming wave will propagate and therefore scatter. When the input beam enters the medium at the correct angle, the scattered light will constructively interfere and create a strong diffracted beam. This effect is similar to the Bragg Scattering that occurs with X-ray reflections. For this reason, AOM often are often referred to as Bragg cells.
Rise Time and Modulation Rate
Two important factors in AOM optimization are rise time and modulation rate. The rise time is considered the time it takes for the acoustic wave to traverse the input beam. This time determines the rate at which the AOM can respond to the signal from the driver, and therefore the rate at which the AOM can modulate the beam. The rise time is dependent on the diameter of the incoming beam and the velocity of the input sound wave. Typical rise time for a one millimeter diameter laser beam is about 150 nanoseconds. An extremely focused beam with a high-frequency sound wave, however, can produce a rise time as low as 4 nanoseconds. AOM with a lower frequency are not limited by input beam diameter. These devices are then best for large bandwidth signals.
Applications
Deflection
When perfectly aligned, AOM are able to diffract about 80% of the incident light into a strong, first-order diffracted beam. We achieve this deflection by turning the acoustic energy source (such as a signal generator) on and off. AOM are able to deflect light from the incident beam in a very short time period. We can also change the diffraction angle by changing the frequency of the input acoustic wave. Within research labs, AOM are especially useful in changing the position of an incoming beam without having to adjust the other optical elements within an experiment. Shifting the beam rapidly is especially useful in laser printers and other laser display devices. Controlled laser beam deflection is also widely used in military and industrial applications here fast steering and control are important.
Holography
Multi-channel AOM are effectively used in an optical setup for holographic purposes. Multi-channel devices modulate or deflect multiple beams at once with an array of transducers. While photographs are a two-dimensional record of light intensity, holographs are recordings of both intensity and phase. This application of phase allows us to reconstruct images in three-dimensions. In order to construct a hologram using an AOM, the electrical holographic information first converts to an acoustic wave. That wave is then sent through the crystal medium within the cell where it meets an optical beam traveling perpendicularly. The exiting beam modulates with a phase-difference pattern across its width. This beam creates a fringe pattern which then projects back onto the optical beam. Thus, a hologram is produced: an encoding of the light field as an interference pattern.
Holograms are often thought of as cool and futuristic images, but they also carry immense data storage capability. These system hold data along all three dimensions with each piece of data measuring about the size of one wavelength of light. Each extremely small piece of data is projected onto a much thicker medium such as a crystalline structure, thus providing an extremely high potential information density. Holographic storage technology is a steadily emerging field with possibilities of long lasting compact systems able to hold high density information. We see basic applications of holographic storage in CDs and DVDs, but modern advancements in holographic technology seek to exceed current limits in storage capacity. Researchers at the University of Southampton r